Genetics: The Fundamentals of Science Biology
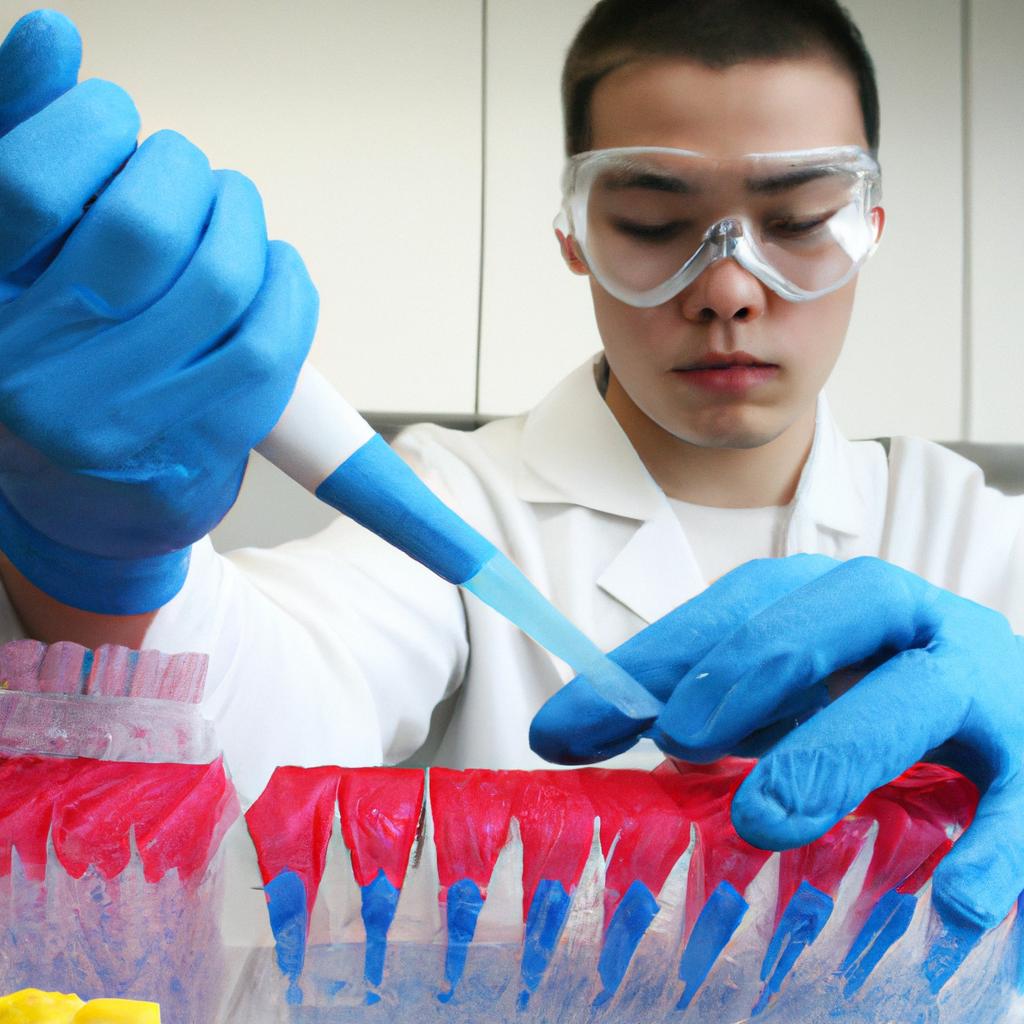
Genetics is a fundamental discipline in the field of biology that explores the inheritance and variation of traits in living organisms. By understanding the mechanisms behind heredity, scientists are able to uncover how genetic information is passed down from one generation to another, providing valuable insights into both the diversity and unity of life on Earth. Through careful study and analysis, researchers have unraveled numerous mysteries surrounding genetics, shedding light on essential concepts such as gene structure, DNA replication, and genetic mutations.
One captivating example that illustrates the significance of genetics can be found in the case study of Gregor Mendel’s experiments with pea plants. In the mid-19th century, Mendel meticulously observed patterns of inheritance by cross-breeding different varieties of peas. His groundbreaking work laid the foundation for modern genetics and led to the discovery of principles like dominant and recessive traits, as well as the concept of segregation during gamete formation. These findings revolutionized our understanding of hereditary processes and paved the way for subsequent advancements in molecular genetics.
To delve deeper into this fascinating field, it is crucial to grasp key fundamentals that underpin genetics. This article aims to explore these basics comprehensively while emphasizing their importance in unraveling complex biological phenomena. By examining topics such as genes, chromosomes , and genetic inheritance, we can gain a better understanding of how genetic information is organized and transmitted within living organisms.
Genes are segments of DNA (deoxyribonucleic acid) that contain the instructions for building and maintaining an organism. They serve as the blueprint for traits such as eye color, height, and susceptibility to certain diseases. Genes are located on structures called chromosomes, which are thread-like strands of DNA found in the nucleus of cells. Humans typically have 46 chromosomes arranged in 23 pairs.
During reproduction, genetic information is passed from parent to offspring through a process called genetic inheritance. This occurs through the formation of reproductive cells called gametes (sperm and eggs), which contain half the number of chromosomes found in other body cells (called somatic cells). When gametes combine during fertilization, the resulting offspring inherit a unique combination of genes from both parents.
Inheritance patterns can be influenced by various factors, including dominant and recessive traits. Dominant traits are expressed when at least one copy of a gene is present, while recessive traits require two copies for expression. For example, if a person inherits one dominant allele for brown eyes from one parent and one recessive allele for blue eyes from another parent, they will have brown eyes because brown eye color is dominant over blue.
Another crucial aspect of genetics is genetic mutations. Mutations are alterations or changes in DNA sequences that can occur spontaneously or be induced by external factors like radiation or chemicals. These changes can result in new variations within populations and contribute to evolution.
The study of genetics has far-reaching applications across various scientific disciplines. It plays a vital role in fields such as medicine, agriculture, forensic science, and conservation biology. By understanding the underlying mechanisms governing heredity, scientists can develop innovative therapies for genetic disorders, improve crop yields through selective breeding techniques, solve criminal investigations using DNA profiling methods, and preserve endangered species by analyzing their genetic diversity.
In conclusion, genetics is a fundamental field of biology that explores the inheritance and variation of traits in living organisms. Through the study of genes, chromosomes, and genetic inheritance patterns, scientists have made significant discoveries about how genetic information is transmitted and expressed. This knowledge has profound implications for our understanding of life’s diversity and unity, as well as its practical applications in various scientific disciplines.
The Basics of Inheritance
The Basics of Inheritance
Imagine a young couple eagerly anticipating the birth of their first child. As they wonder about the traits and characteristics their baby will inherit, they unknowingly delve into the fascinating world of genetics. Genetics is the branch of science that studies how traits are passed down from one generation to another through the transmission of genetic information encoded in DNA.
Understanding inheritance requires grasping several key concepts. First and foremost is the idea that our genes determine many aspects of who we are, including physical features like eye color or height, as well as predispositions to certain diseases or conditions. Genes are segments of DNA found on chromosomes within the nucleus of our cells. Each gene carries instructions for building specific proteins necessary for various biological processes.
Inherited traits can be either dominant or recessive. Dominant traits manifest when at least one copy of the corresponding gene is present, while recessive traits require both copies to be inherited. For instance, let’s consider eye color: if one parent has brown eyes (dominant trait) and the other has blue eyes (recessive trait), their child may inherit a combination resulting in brown eyes due to dominance.
To illustrate further, here’s an example bullet point list highlighting some interesting facts about inheritance:
- Genetic variations occur due to mutations in DNA.
- Some traits exhibit incomplete dominance or codominance.
- Environmental factors can influence gene expression.
- Understanding inheritance patterns helps predict risk factors for certain diseases.
Additionally, it might be helpful to visualize this information using a table:
Trait | Dominant Allele | Recessive Allele |
---|---|---|
Eye Color | Brown | Blue |
Hair Type | Straight | Curly |
Blood Type | A | O |
Thumb Shape | Hitchhiker’s thumb | No hitchhiker’s |
As we delve deeper into the fascinating world of genetics, our next focus will revolve around understanding DNA replication. This process is essential for the transmission and conservation of genetic information from one generation to another.
In summary, by uncovering the basics of inheritance, we gain insight into how genes shape our physical attributes and predispositions. Through dominant and recessive traits, mutations in DNA, and gene expression influenced by environmental factors, we can better understand the complex interplay between genetics and individual characteristics. With this foundation laid, let’s now explore the intricate process of DNA replication and its significance in maintaining inherited traits.
Understanding DNA Replication
Building upon our understanding of the basics of inheritance, let us now delve deeper into the intricate process of DNA replication.
DNA replication is a fundamental mechanism that ensures genetic information is accurately transmitted from one generation to the next. To illustrate this process, imagine a scenario where an individual undergoes cell division in order to produce new cells for growth and repair. During DNA replication, each double-stranded DNA molecule unwinds and separates into two complementary strands. These strands then act as templates for the synthesis of new DNA molecules with identical sequences.
To comprehend the intricacies of DNA replication, it is essential to understand several key components and processes involved:
- Helicase: This enzyme plays a crucial role in unwinding the double helix structure by breaking hydrogen bonds between base pairs.
- Primase: As DNA cannot begin synthesizing without a primer, primase initiates replication by synthesizing short RNA primers that serve as starting points for DNA polymerases.
- Leading Strand: The leading strand is synthesized continuously, while its counterpart—the lagging strand—is formed discontinuously in small fragments called Okazaki fragments.
- Ligase: Once all these fragments are synthesized on the lagging strand, ligase helps join them together into a continuous chain.
- Discovering the astounding precision behind every step of this complex process evokes awe at nature’s ability to sustain life through such intricacy.
- Understanding how slight errors during replication can lead to mutations highlights both the vulnerability and resilience of living organisms.
- Realizing that human beings share more than 99% of their genetic material emphasizes our interconnectedness as a species.
- Recognizing that even minor variations within this highly conserved process contribute to genetic diversity sparks curiosity about evolution’s driving forces.
Process | Enzyme | Function |
---|---|---|
Unwinding | Helicase | Separates the DNA strands |
Priming | Primase | Synthesizes RNA primers |
Leading Strand | DNA Polymerase III | Synthesizes the leading strand continuously |
Lagging Strand | DNA Polymerase I and Ligase | Synthesize Okazaki fragments and join them into a continuous chain |
As we continue to explore the wonders of genetics, our journey will now take us towards unraveling the mechanisms of gene expression. Understanding these intricate processes is crucial in comprehending how genetic information manifests itself within an organism’s phenotype.
Unraveling the Mechanisms of Gene Expression
Understanding DNA Replication: Unveiling the Mechanisms
Imagine a scenario where a group of scientists embarked on an ambitious quest to understand the intricate process of DNA Replication. Through their rigorous research, they uncovered fascinating insights into this fundamental biological phenomenon. In this section, we will delve deeper into these breakthroughs and explore the mechanisms behind DNA replication.
To comprehend the intricacies of DNA replication, it is important to grasp several key aspects:
- Helicase Unwinding: An essential first step in DNA replication involves helicase enzymes unwinding the double-stranded DNA molecule, separating its two strands.
- Priming with RNA: Once the strands are separated, primases come into play by synthesizing short RNA segments called primers along both strands.
- DNA Polymerase Elongation: The leading strand serves as a template for continuous synthesis by DNA polymerase III, while the lagging strand undergoes discontinuous synthesis forming Okazaki fragments.
- Ligase Sealing: Finally, ligase seals any gaps between adjacent fragments by catalyzing phosphodiester bond formation.
Now let us consider an emotionally evocative example that highlights the significance of understanding DNA replication in real-world scenarios. Imagine a patient diagnosed with a rare genetic disorder caused by errors during DNA replication. By unraveling the underlying mechanisms and identifying potential points of failure in this process, researchers can work towards developing targeted therapies or preventive measures to alleviate suffering and improve lives.
In addition to textual explanation, visual aids such as tables can aid comprehension and emotional engagement within scientific discourse. Consider the following table showcasing key players involved in various stages of DNA replication:
Stage | Key Player |
---|---|
Helicase unwinding | MCM Complex |
Priming with RNA | Primase |
Leading Strand Synthesis | DNA Polymerase III |
Lagging Strand Synthesis | DNA Polymerase III |
Ligase Sealing | DNA Ligase |
As we conclude this section, the knowledge gained from understanding DNA replication not only expands our scientific understanding but also has considerable implications for human health. Exploring how errors during replication can lead to genetic disorders will be the focus of the subsequent section, “Exploring the Causes of Genetic Disorders.” By gaining insights into these causes, we can strive towards better prevention and treatment strategies, offering hope to those affected by such conditions.
Transition Sentence: Building upon our understanding of DNA replication mechanisms, let us now transition into exploring the causes of genetic disorders.
Exploring the Causes of Genetic Disorders
Unveiling the Complexities of Genetic Inheritance
Imagine a young couple eagerly anticipating the arrival of their first child. As they prepare for this life-changing event, they may wonder what traits their baby will inherit from each parent. Will the child have their father’s curly hair or their mother’s blue eyes? These questions can be answered by delving into the fascinating world of genetic inheritance.
Genetic inheritance is the process through which traits are passed down from one generation to another. It involves the transmission of genes, segments of DNA that carry instructions for building and functioning organisms. To understand this intricate process, we must explore its underlying mechanisms and factors that contribute to genetic variation.
Firstly, Gene Expression plays a pivotal role in determining an organism’s characteristics. Genes can be activated or silenced depending on various regulatory factors within cells. Understanding how these mechanisms operate allows scientists to unravel complex biological processes such as development and disease progression.
Secondly, it is crucial to investigate the causes behind genetic disorders. Some conditions arise due to mutations in specific genes, leading to abnormal protein production or function. Identifying these mutations provides valuable insights into disease pathology and aids in developing targeted therapies.
To grasp the significance of genetic inheritance fully, consider the following emotional responses:
- Awe: The intricacy with which our bodies pass down traits across generations leaves us in awe of nature’s complexity.
- Curiosity: Unraveling the mysteries of genetics stimulates curiosity about our own unique makeup and those around us.
- Empathy: Awareness of genetic disorders evokes empathy for individuals and families affected by these conditions.
- Hope: Advances in understanding genetic inheritance bring hope for improved treatments and preventative measures.
Trait | Mode of Inheritance | Example |
---|---|---|
Eye color | Polygenic | Brown (dominant) |
Blood type | Multiple alleles | AB (codominant) |
Huntington’s Disease | Autosomal dominant | Presence of huntingtin gene |
In conclusion, the study of genetic inheritance unravels a tapestry that connects generations and shapes our individuality. By exploring the mechanisms behind gene expression and investigating the causes of Genetic Disorders, we gain valuable insights into the complexities of life. Understanding these intricacies evokes emotions such as awe, curiosity, empathy, and hope.
Transitioning to “The Importance of Genetic Variation,” we realize that genetics not only impacts individuals but also influences entire populations through its role in evolution.
The Importance of Genetic Variation
Genetic disorders can arise from a variety of factors, including mutations in DNA sequences, chromosomal abnormalities, and inheritance patterns. To illustrate this concept further, let us consider the case study of Sarah, a 35-year-old woman who has recently been diagnosed with Huntington’s disease—a neurodegenerative disorder characterized by involuntary movements, cognitive decline, and psychiatric symptoms.
Firstly, it is essential to understand that genetic disorders can result from mutations in specific genes. In Sarah’s case, she inherited an altered form of the huntingtin gene from her father. This mutation causes an abnormal repetition of certain nucleotides within the gene sequence, leading to the production of a faulty protein. As a consequence, nerve cells gradually degenerate over time, causing the characteristic symptoms associated with Huntington’s disease.
Furthermore, chromosomal abnormalities can also contribute to genetic disorders. For instance, Down syndrome occurs when there is an additional copy of chromosome 21 due to nondisjunction during cell division. This extra genetic material alters the normal development and functioning of various organ systems in affected individuals.
To highlight different types and causes of genetic disorders:
- Spontaneous mutations: These occur randomly during DNA replication or as a result of environmental factors such as radiation exposure.
- Autosomal recessive inheritance: A disorder manifests only when both copies of a particular gene are mutated; carriers may not exhibit any symptoms but have one mutated copy.
- X-linked inheritance: Certain conditions are more prevalent in males because they inherit one X chromosome (from their mother) carrying the mutation.
- Multifactorial inheritance: Complex interactions between multiple genes and environmental factors contribute to these disorders’ development.
Type | Example | Prevalence |
---|---|---|
Single-gene disorders | Cystic fibrosis | 1 in every 2,500 births |
Chromosomal disorders | Turner syndrome | 1 in every 2,500 females |
Autosomal recessive | Sickle cell anemia | Most common in populations with a history of malaria |
X-linked | Hemophilia | Affects approximately 1 in 5,000 males |
In summary, understanding the causes of genetic disorders is vital for effective diagnosis, treatment, and prevention strategies. These conditions can arise from mutations within specific genes or chromosomal abnormalities inherited through various patterns. By studying individual cases like Sarah’s and exploring different types of genetic disorders and their prevalence rates, scientists gain valuable insights into the complexities of human genetics.
Transitioning to the subsequent section about “Unlocking the Secrets of Epigenetics,” researchers continue to delve deeper into the intricate mechanisms that influence gene expression and its potential impact on health outcomes.
Unlocking the Secrets of Epigenetics
Section H2: ‘Exploring the Mechanisms of Inheritance’
Building upon our understanding of genetic variation, let us now delve into the intricate mechanisms through which genetic traits are inherited. To illustrate this concept, consider a hypothetical scenario where two individuals with different eye colors – one with blue eyes and another with brown eyes – have a child. This offspring inherits one copy of the gene responsible for eye color from each parent, resulting in a unique combination that determines their own eye color.
In exploring how genes are passed down from generation to generation, several key factors come into play:
-
Mendelian Inheritance Patterns: Gregor Mendel’s groundbreaking work laid the foundation for our understanding of inheritance patterns. By studying pea plants, he discovered that certain traits follow predictable patterns of transmission from parents to offspring. These patterns include dominant-recessive relationships and segregation during gamete formation.
-
Genetic Recombination: The process of genetic recombination occurs during meiosis when chromosomes exchange segments of DNA. This mechanism ensures genetic diversity within populations by shuffling alleles between homologous chromosomes and creating new combinations of genetic material.
-
Sex-Linked Inheritance: Some traits are linked to specific sex chromosomes (typically X or Y). For example, hemophilia is an X-linked recessive disorder primarily affecting males due to its presence on the X chromosome. Understanding these sex-linked inheritance patterns is crucial in diagnosing and managing such conditions.
-
Non-Mendelian Inheritance: While Mendelian genetics provide a solid framework, not all traits adhere strictly to his laws. Non-Mendelian inheritance includes phenomena like incomplete dominance, codominance, multiple alleles, and polygenic inheritance – all contributing to the complexity and diversity observed in human phenotypes.
Inheritance Pattern | Description |
---|---|
Dominant-Recessive | Traits controlled by dominant or recessive alleles |
Codominance | Both alleles contribute to the phenotype, resulting in a blended or combined trait |
Incomplete Dominance | Heterozygous individuals display an intermediate phenotype |
Sex-Linked | Traits associated with sex chromosomes, leading to differences in inheritance between males and females |
In summary, understanding the mechanisms of inheritance is crucial for comprehending how genes are transmitted across generations. Through Mendelian patterns, genetic recombination, and non-Mendelian phenomena, individuals inherit traits that shape their physical characteristics and predispositions to certain conditions. By exploring these intricate processes, we gain insights into the complexity and diversity of inherited traits.
Moving forward from this exploration of inheritance mechanisms, our focus now shifts towards examining specific patterns observed in inherited traits.
Patterns of Inherited Traits
As we delve further into the intricate world of genetics, we encounter the fascinating concept of epigenetics. Epigenetic modifications refer to changes in gene expression that occur without altering the underlying DNA sequence. To illustrate this phenomenon, let us consider a hypothetical case study involving identical twins with contrasting physical traits despite their shared genetic makeup.
In our case study, Sarah and Emily are identical twins who possess the same genes for eye color. However, Sarah has brown eyes while Emily has blue eyes. This apparent contradiction can be explained by Epigenetic Modifications. It is likely that certain environmental factors or experiences have influenced the activation or silencing of specific genes related to eye pigmentation in each twin, resulting in different phenotypic outcomes.
Understanding epigenetic modifications provides valuable insights into how inherited traits can manifest differently within individuals carrying similar genetic information. These modifications play a crucial role in numerous biological processes, including embryonic development, aging, and disease susceptibility. By unraveling these mechanisms, scientists aim to decipher how various external factors interact with our genome to shape our individual characteristics.
To grasp the significance of epigenetics fully, here are some key points to ponder:
- Epigenetic modifications can be reversible and dynamic.
- Environmental factors such as diet, stress levels, exposure to toxins, and lifestyle choices can influence gene expression through epigenetic alterations.
- The effects of epigenetic modifications can extend beyond an individual’s lifetime; they may be passed down from one generation to another.
- Epigenetics serves as a vital bridge linking nature (genetics) and nurture (environmental influences), highlighting the complex interplay between these two factors.
Let us now explore another fundamental aspect of genetics – the replication process of genetic material – which elucidates how accurate transmission occurs during cell division while safeguarding against errors and mutations.
The Replication Process of Genetic Material
In the previous section, we explored the fascinating world of patterns of inherited traits. One example that sheds light on this concept is the case study of a family where several members have an inherited condition called Huntington’s disease. This neurodegenerative disorder is caused by a mutation in the huntingtin gene, leading to progressive cognitive and motor decline.
Understanding how traits are passed down from one generation to another is essential in genetics. It allows us to comprehend the underlying mechanisms responsible for variations in physical characteristics, behaviors, and susceptibility to certain diseases. Here are four key points to consider when examining patterns of inherited traits:
- Mendelian inheritance: Gregor Mendel’s experiments with pea plants laid the foundation for our understanding of heredity. His laws describe predictable patterns of inheritance based on dominant and recessive alleles.
- Polygenic inheritance: Not all traits can be explained by simple Mendelian genetics. Some characteristics arise due to interactions between multiple genes, known as polygenic inheritance. Examples include height, skin color, and intelligence.
- Codominance and incomplete dominance: In some cases, neither allele is completely dominant or recessive over the other. When both alleles contribute equally to the phenotype, it is called codominance (e.g., blood type). Incomplete dominance occurs when a heterozygous individual displays an intermediate phenotype (e.g., pink flowers resulting from crossing red and white flower parents).
- Sex-linked traits: Certain traits are associated with genes located on sex chromosomes. For instance, hemophilia A is more common in males because it is linked to a defective X chromosome.
To further enhance your understanding of these concepts, let’s take a look at the table below depicting different types of inheritance:
Type of Inheritance | Description | Example |
---|---|---|
Autosomal Dominant | Trait expressed if present | Huntington’s disease |
Autosomal Recessive | Trait expressed if homozygous | Cystic fibrosis |
X-Linked Dominant | Trait expressed in males | Hypophosphatemic rickets |
X-Linked Recessive | More common in males | Color blindness, hemophilia A |
As we delve deeper into the intricacies of genetics, it becomes evident that patterns of inherited traits play a crucial role in shaping who we are. By understanding these patterns and their underlying mechanisms, we can gain insights into human diversity and the development of genetic disorders.
Transitioning to our next section on “The Replication Process of Genetic Material,” let us now explore how genes are regulated within cells.
Regulation of Gene Activity
Section Title: The Role of Epigenetics in Gene Regulation
Building upon our understanding of the replication process, it is essential to delve into the intricate mechanism that regulates gene activity – epigenetics. By exploring how external factors influence gene expression, we will gain valuable insight into the complexity and adaptability of living organisms.
Epigenetic modifications can be likened to a symphony conductor fine-tuning an orchestra’s performance. Consider this hypothetical case study: identical twins with similar genetic material but distinct physical appearances due to varied environmental exposures throughout their lives. These differences are attributed to epigenetic changes that occur without altering the underlying DNA sequence. Such modifications allow genes to be switched on or off in response to cues from the environment, resulting in diverse phenotypic outcomes.
Understanding epigenetics involves recognizing key concepts:
- DNA methylation: A chemical modification where methyl groups attach themselves to specific regions of DNA, silencing gene expression.
- Histone modification: Alterations made to histone proteins around which DNA is wrapped, impacting its accessibility for transcription factors.
- Non-coding RNAs: Small RNA molecules that regulate gene expression by blocking translation or promoting mRNA degradation.
- Chromatin remodeling complexes: Enzymes responsible for changing chromatin structure and thus influencing gene accessibility.
To provide a visual representation of these concepts, here is a table showcasing different types of epigenetic modifications and their effects:
Epigenetic Modification | Effect on Gene Expression |
---|---|
DNA Methylation | Silences gene expression |
Histone Modification | Alters accessibility |
Non-coding RNAs | Regulates mRNA function |
Chromatin Remodeling | Changes gene accessibility |
Recognizing the role played by epigenetics expands our comprehension of genetic regulation beyond simple inheritance patterns. It highlights how external influences shape an organism’s phenotype and adaptability to changing environments. By unlocking the mechanisms underlying epigenetic modifications, we gain a deeper appreciation for the complexity of life.
Transition into subsequent section:
Identifying and Managing Genetic Disorders necessitates a thorough understanding of genetic regulation, including both replication processes and epigenetics. Through this exploration, we will uncover the potential applications of our knowledge in improving human health and well-being.
Identifying and Managing Genetic Disorders
Section H2: Regulation of Gene Activity
Building upon our understanding of gene regulation, we now delve into the critical task of identifying and managing genetic disorders. To illustrate the importance of this topic, let us consider a hypothetical case study involving Sarah, a 30-year-old woman who has been experiencing unexplained health issues since her teenage years. Through extensive genetic testing and analysis, it was discovered that Sarah carries a mutation in a key regulatory gene responsible for glucose metabolism. This finding shed light on the underlying cause of her symptoms and paved the way for targeted interventions.
Managing Genetic Disorders:
-
Early Diagnosis and Screening:
- Timely identification through newborn screening programs allows for early intervention.
- Comprehensive diagnostic tests such as DNA sequencing can be employed to detect genetic abnormalities.
- Non-invasive prenatal testing enables detection of certain genetic disorders during pregnancy.
- Carrier screening aids in family planning by assessing the risk of passing on specific conditions.
-
Treatment Strategies:
- Pharmacological interventions aim to alleviate symptoms or slow disease progression.
- Gene therapy offers promising avenues to correct faulty genes or introduce functional ones.
- Stem cell transplantation shows potential in replacing damaged cells with healthy ones.
- Nutritional management plays a crucial role in mitigating the impact of certain metabolic disorders.
-
Supportive Care and Counseling:
- Psychological support helps individuals and families cope with emotional challenges associated with genetic disorders.
- Genetic counseling provides information about risks, inheritance patterns, and available options.
- Patient advocacy groups offer invaluable resources, guidance, and community support networks.
- Empathy
- Hope
- Fear
- Resilience
Emotional Table:
Emotion | Description |
---|---|
Empathy | Understanding and sharing the feelings of those affected |
Hope | The belief in positive outcomes despite adversity |
Fear | An emotional response to the uncertainty of genetic disorders |
Resilience | The ability to bounce back and adapt in the face of challenges |
Recognizing the impact that genetic disorders have on individuals and society, it is essential to understand how mutations contribute to genetic variation. This brings us to our next section – “The Role of Mutation in Genetic Variation” where we explore this fascinating aspect further.
[End of Section H2]
Section H2: Identifying and Managing Genetic Disorders
The Role of Mutation in Genetic Variation
Section: The Role of Mutation in Genetic Variation
In the previous section, we explored how genetic disorders can be identified and managed. Now, let us delve into the fascinating world of mutations and their crucial role in creating genetic variation. To illustrate this concept, consider a hypothetical scenario where two individuals with different hair colors have a child who inherits a unique combination of both parents’ traits.
Mutations are alterations that occur in an organism’s DNA sequence, leading to changes in its genetic makeup. These variations can arise spontaneously or as a result of external factors such as radiation exposure or chemical damage. One example is the mutation responsible for changing the pigmentation gene in our hypothetical family’s offspring, resulting in distinct hair color not present in either parent.
Understanding the significance of mutations requires recognizing their impact on genetic diversity. Here are four key points to contemplate:
- Mutations drive evolution by introducing new genetic variants.
- Some mutations may confer advantages, increasing an organism’s fitness.
- Others may be detrimental and reduce chances of survival or reproduction.
- Neutral mutations have no noticeable effect but accumulate over time.
To comprehend the wide array of effects caused by mutations, it is essential to examine specific instances across various species. Consider the following table showcasing selected examples from nature:
Species | Mutation Effect |
---|---|
Fruit Fly | Increased resistance to insecticides |
Cattle | Enhanced milk production |
Bacteria | Antibiotic resistance |
Humans | Sickle cell trait causing disease symptoms |
This brief overview demonstrates that while some mutations might bring benefits like increased resistance or enhanced abilities, others can lead to adverse consequences such as disease susceptibility. Ultimately, these diverse outcomes contribute significantly to shaping the intricate tapestry of life on Earth.
Moving forward, we will explore yet another captivating aspect within genetics – epigenetic modifications and gene regulation. Understanding how genes are regulated provides valuable insights into the complex mechanisms that guide cellular processes and development.
Now, let us venture into the realm of epigenetic modifications and gene regulation.
Epigenetic Modifications and Gene Regulation
Building upon the understanding of genetic variation through mutation, this section explores another crucial aspect of genetics—epigenetic modifications and gene regulation. Epigenetics refers to changes in gene expression or cellular phenotype that occur without alterations to the DNA sequence itself. These modifications can be influenced by various factors such as environmental conditions, lifestyle choices, and even early-life experiences.
To illustrate the impact of epigenetic modifications, consider a hypothetical case study involving identical twins raised in different environments. Twin A, growing up in an urban area with high air pollution levels, may experience altered gene expression patterns compared to Twin B who was raised in a rural environment with cleaner air. This difference arises due to epigenetic modifications induced by exposure to environmental pollutants, highlighting how external factors can influence genetic traits.
One key mechanism involved in epigenetic modifications is DNA methylation—a process where methyl groups are added to specific regions of the DNA molecule. This modification often leads to gene silencing, restricting their activity and preventing them from being expressed. Conversely, some genes may undergo demethylation, allowing for increased expression. Apart from DNA methylation, histone modifications also play a significant role in regulating gene expression by altering the accessibility of genes within chromatin structures.
Understanding the complexity of epigenetics requires considering multiple aspects:
- Environmental influences on epigenetic marks
- Interplay between genetic and epigenetic variations
- Long-term effects of these modifications across generations
- Therapeutic potential for targeting epigenetic mechanisms
Table 1 showcases examples of diseases known to have an association with abnormal epigenetic patterns:
Disease | Abnormal Epigenetic Pattern |
---|---|
Cancer | Hypermethylation |
Neurodevelopmental disorders | Hypomethylation |
Cardiovascular diseases | Histone modifications |
These examples highlight the broad impact of epigenetic modifications on human health and disease. By exploring how gene regulation can be influenced by factors beyond DNA sequence, scientists strive to unravel the intricate interplay between genetics and environmental influences.
In summary, this section has shed light on the fascinating world of epigenetic modifications and their role in regulating gene expression patterns. The hypothetical case study and discussion around key mechanisms provide insight into how external factors can shape genetic traits through epigenetics. Understanding these complex processes offers promising avenues for further research into both normal development and various diseases affected by abnormal epigenetic patterns.