DNA Replication: The Genetic Code in Science Biology
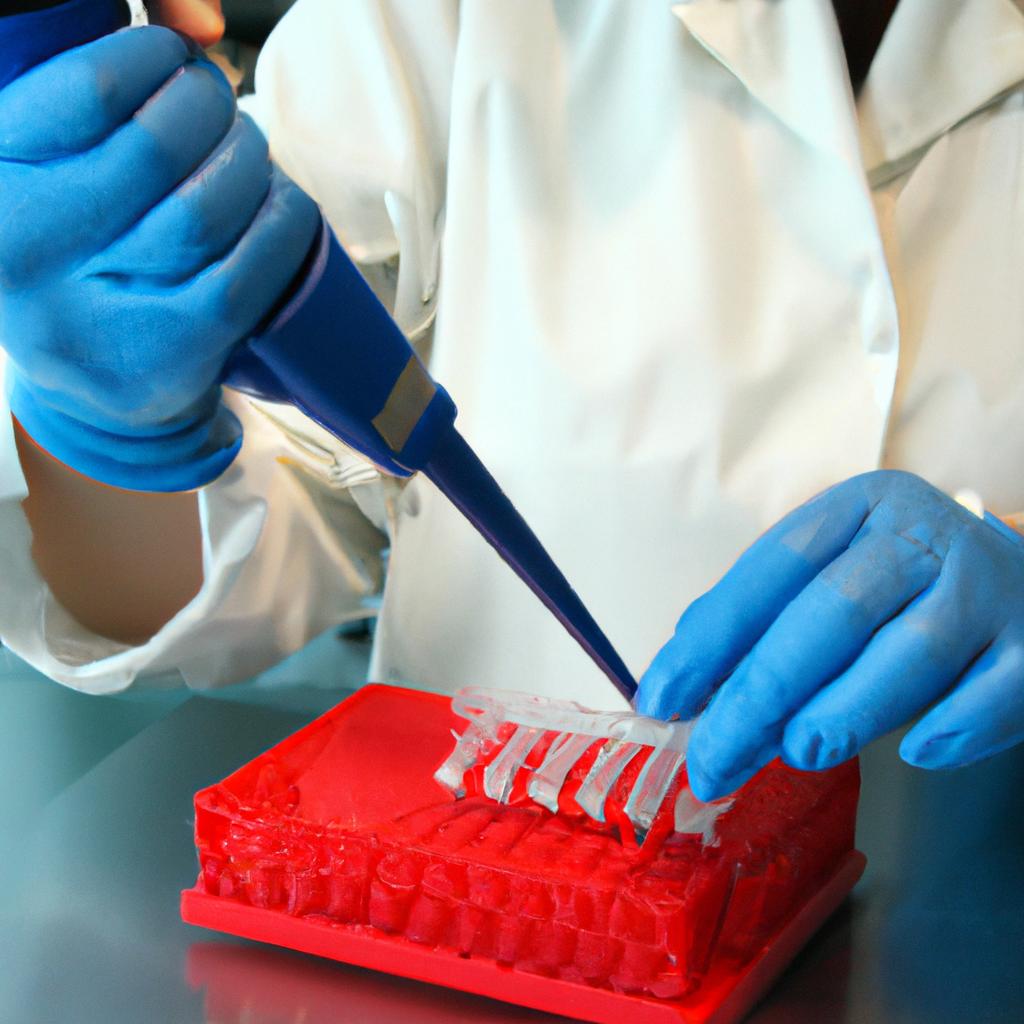
DNA replication is a fundamental process that plays a crucial role in the transmission of genetic information from one generation to the next. It occurs prior to cell division, ensuring that each daughter cell receives an accurate copy of the parent’s DNA. This intricate mechanism involves multiple enzymes and proteins working together in a highly coordinated manner. As scientists delve deeper into understanding DNA replication, they uncover fascinating insights into how genetic information is faithfully replicated and maintained.
Imagine a scenario where errors occur during DNA replication. These mistakes could lead to mutations and alterations in the genetic code, potentially resulting in adverse health conditions or developmental disorders. Therefore, studying DNA replication not only provides valuable knowledge about basic cellular processes but also has significant implications for fields such as medicine and genetics research.
In this article, we will explore the intricacies of DNA replication, its importance in maintaining genetic fidelity, and the various mechanisms involved. By delving into this captivating subject matter, we can gain a better understanding of how our genes are perpetuated through generations and appreciate the complex machinery responsible for accurately duplicating our genetic code.
The Structure of DNA
Did you know that the discovery of the structure of DNA has revolutionized our understanding of genetics and paved the way for numerous scientific advancements? Take, for example, the case study of Rosalind Franklin whose work on X-ray diffraction images played a crucial role in unraveling the double helix structure. Understanding the structure of DNA is fundamental to comprehending how genetic information is stored and transmitted.
To begin with, DNA (deoxyribonucleic acid) consists of two antiparallel strands twisted around each other to form a double helix. Each strand is composed of nucleotides which consist of a sugar molecule, phosphate group, and nitrogenous base. The two strands are held together by hydrogen bonds between complementary bases: adenine pairs with thymine (A-T), while cytosine pairs with guanine (C-G). This specific pairing ensures faithful replication during cell division and accurate transfer of genetic information from one generation to the next.
Furthermore, DNA possesses several key features that contribute to its stability and functionality:
- Double-stranded: The double-helix structure provides stability and protection for the genetic material.
- Anti-parallel orientation: The two strands run in opposite directions, allowing efficient replication through semi-conservative synthesis.
- Complementary base pairing: Adenine always pairs with thymine, and cytosine always pairs with guanine. This specificity allows precise copying and transmission of genetic information.
- Supercoiling: DNA can twist upon itself due to supercoiling, enabling it to fit within the confined space inside cells.
Features | Description |
---|---|
Double-stranded | Provides stability and protection |
Anti-parallel | Allows efficient replication |
Complementary | Enables precise copying and transmission |
Supercoiling | Facilitates compact packaging within cells |
Understanding the structure of DNA not only sheds light on its remarkable stability and functionality but also provides a foundation for comprehending how genetic information is precisely replicated, ensuring the accurate transmission of traits from one generation to another. In the subsequent section about “The Process of DNA Replication,” we will explore this intricate process in detail, delving into the mechanisms by which cells duplicate their DNA molecules.
Now that we have established the structural basis of DNA, let us delve into the fascinating world of DNA replication.
The Process of DNA Replication
Transitioning seamlessly from our previous discussion on the structure of DNA, let us now delve into the intricate process of DNA replication. To illustrate its significance, consider a hypothetical scenario where a cell is undergoing rapid division to heal a wound. In this case, accurate and efficient DNA replication becomes crucial to ensure that each new cell receives an exact copy of the genetic material necessary for proper functioning.
DNA replication involves several key steps that work together harmoniously to produce two identical copies of the original DNA molecule. These steps can be summarized as follows:
- Initiation: This marks the beginning of the replication process, where specific proteins recognize and bind to particular sequences on the DNA strand called origins of replication. These proteins then unwind and separate the double helix, creating a region known as the replication fork.
- Elongation: Once initiation has occurred, specialized enzymes called DNA polymerases catalyze the addition of nucleotides complementary to those present on each separated strand. As these nucleotides are added one by one, they form new strands alongside the existing ones.
- Termination: As elongation progresses along both strands at the replication fork, it eventually reaches termination sites or regions that signal completion. Here, various mechanisms come into play to halt further synthesis and ensure accuracy in copying.
In contemplating the intricacies of DNA replication, we begin to grasp just how remarkable this cellular process truly is. Imagine millions upon millions of cells dividing within our bodies every day—their ability to faithfully replicate their genetic material astounds us all. Reflecting on this awe-inspiring phenomenon allows us to appreciate nature’s ingenuity in perpetuating life itself.
As we explore further into understanding DNA replication, we will next focus on the enzymes involved in orchestrating this complex process with precision and efficiency. By delving deeper into their functions and interactions within the cellular machinery, we can gain profound insights into the remarkable world of genetic replication and its implications in biological science.
Next section: Enzymes Involved in DNA Replication
Enzymes Involved in DNA Replication
Imagine a scenario where the process of DNA replication goes awry, resulting in errors and mutations. Such inaccuracies can have serious consequences for an organism’s survival and development. One notable example is the disorder known as Xeroderma Pigmentosum (XP), a rare genetic condition characterized by extreme sensitivity to sunlight due to defective DNA repair mechanisms. Individuals with XP are unable to efficiently correct mistakes that occur during DNA replication, leading to an increased risk of skin cancer and other associated health problems.
To understand the significance of accurate DNA replication, let us delve into some key factors that contribute to its importance:
-
Genetic Stability: Accurate DNA replication ensures the faithful transmission of genetic information from one generation to the next. Without precise replication, there would be a high likelihood of introducing harmful mutations or deleting essential genes, which could disrupt normal cellular function.
-
Evolutionary Adaptation: Through accurate replication, organisms can accumulate beneficial changes over time, allowing them to adapt and survive in changing environments. This process drives evolution by preserving advantageous traits while eliminating detrimental ones.
-
Cellular Function: Proper functioning of cells relies on accurate duplication of their genetic material during each cell division cycle. Mistakes in DNA replication can lead to dysfunctional proteins, impaired signaling pathways, and overall disruption of cellular processes necessary for growth and development.
The importance of maintaining accuracy during DNA replication cannot be overstated. To emphasize this point further, consider the following table showcasing various disorders caused by errors in DNA replication:
Disorder | Symptoms | Consequences |
---|---|---|
Bloom Syndrome | Short stature, sun-sensitive skin | Increased susceptibility to cancers |
Werner Syndrome | Premature aging | Higher incidence of age-related diseases |
Fanconi Anemia | Bone marrow failure | Predisposition to leukemia and solid tumors |
Ataxia-Telangiectasia | Neurological abnormalities, weakened immunity | Increased risk of cancer, neurodegenerative disorders |
As we can see from these examples, accurate DNA replication is crucial for maintaining genetic integrity and preventing a wide variety of disorders. In the subsequent section, we will explore the intricate processes occurring at the replication fork and within replication bubbles.
With an understanding of the importance of accurate DNA replication in mind, let us now turn our attention to the intricacies of the replication fork and replication bubble.
Replication Fork and Replication Bubble
In the previous section, we explored the crucial role of enzymes in DNA replication. Now, let us delve further into this intricate process by examining the replication fork and replication bubble. To better understand these concepts, consider a hypothetical scenario involving a cell undergoing DNA replication.
Imagine a cell dividing to produce two identical daughter cells. As part of this process, the double-stranded DNA molecule needs to be replicated accurately. This is achieved through the coordinated action of several enzymes that are specifically involved in different stages of DNA replication.
Firstly, helicase unwinds the double helix structure at specific sites called origins of replication. Once separated, single-strand binding proteins stabilize the unpaired DNA strands and prevent them from re-annealing. At this point, primase synthesizes short RNA sequences known as primers which serve as starting points for DNA synthesis.
Now comes one of the key players – DNA polymerase. It adds nucleotides to the growing strand using complementary base pairing with each original template strand. However, only in one direction (5′ to 3′) can it synthesize continuously along the leading strand. For the lagging strand, discontinuous synthesis occurs in small fragments called Okazaki fragments due to its antiparallel orientation.
To illustrate some important aspects visually:
- Replication Fork: In our hypothetical scenario, imagine the separation of parental strands forming a Y-shaped structure called a replication fork.
- Helicase: Helicase enzyme acts like an unwinding zipper on either side of this fork.
- Single-strand Binding Proteins: These proteins keep the separated strands apart until they can be copied.
- Primase: Primase lays down RNA primers necessary for initiating new strands during later steps.
Let’s summarize what we have learned so far without stating “Finally” or “In conclusion.” The enzymes involved in DNA replication work together in a coordinated manner to ensure the accurate duplication of genetic information. In the subsequent section, we will explore how this process leads to semi-conservative DNA replication.
Semi-Conservative DNA Replication
Replication Bubble and Replication Fork: Key Features of DNA Replication
Imagine a bustling city with multiple construction sites simultaneously erecting new buildings. Each site has workers diligently constructing different sections, all working towards the common goal of completing their structures. Similarly, in DNA replication, the process involves several key features that work together harmoniously to ensure an accurate duplication of genetic information.
One crucial element of DNA replication is the formation of replication bubbles and replication forks. These structures emerge as the double helix unwinds at specific points along the DNA molecule, creating two separate strands known as templates. Within each template strand, a leading and lagging strand are formed due to differences in directionality during synthesis.
The leading strand is synthesized continuously in the same direction as the replication fork moves forward. In contrast, the lagging strand is synthesized discontinuously in small fragments called Okazaki fragments because it runs opposite to the movement of the replication fork. The gaps between these fragments are later filled by another enzyme, resulting in a complete complementary strand.
To better understand this intricate process, consider some key aspects:
- Helicase: This enzyme plays a vital role in unwinding the double-stranded DNA molecule at the origin of replication.
- Primase: This enzyme synthesizes short RNA primers that provide starting points for DNA synthesis on both strands.
- DNA polymerase III: As one of the primary enzymes involved in elongation, it catalyzes the addition of nucleotides onto the growing daughter strands.
- Ligase: After Okazaki fragments have been synthesized on the lagging strand, ligase helps seal any remaining nicks or breaks within the newly formed DNA molecule.
By coordinating these essential components and processes, cells can accurately replicate their genetic material while maintaining its integrity.
Enzyme | Function | Significance |
---|---|---|
Helicase | Unwinds the double-stranded DNA molecule | Facilitates access to template strands |
Primase | Synthesizes RNA primers that provide starting points for synthesis | Initiates DNA replication on both leading and lagging strands |
DNA polymerase III | Catalyzes addition of nucleotides onto growing daughter strands | Ensures accurate duplication of genetic information |
Ligase | Seals any remaining nicks or breaks within the newly formed DNA molecule | Completes the formation of a continuous complementary strand |
These enzymes, with their distinct functions and interplay, enable cells to successfully replicate their DNA. The significance of this process extends beyond mere duplication; it ensures the preservation and transmission of genetic information from one generation to the next.
Transitioning seamlessly into the subsequent section, understanding the features and mechanics behind DNA replication is crucial in comprehending its broader significance in various biological processes such as cell division, development, and heredity.
Significance of DNA Replication
Semi-Conservative DNA Replication is a fundamental process that ensures accurate transmission of genetic information from one generation to the next. In this section, we will explore the significance of DNA replication in maintaining the stability and diversity of life. To illustrate its importance, let us consider the case of a cell undergoing rapid division during embryonic development.
During early stages of embryogenesis, when cells divide rapidly, each new cell receives a copy of the parent cell’s DNA through semi-conservative replication. This process guarantees that each daughter cell possesses an identical set of genetic instructions as the parent cell. For example, if there is a mutation in a specific gene responsible for limb development, all subsequent cells derived from the mutated cell would also carry this faulty gene. Consequently, understanding how errors occur during DNA replication can provide valuable insights into developmental disorders such as congenital limb malformations.
The significance of DNA replication extends beyond individual organisms; it plays a crucial role in evolution by introducing variations within populations. Genetic diversity arises due to several factors associated with DNA replication:
- Mutation: Errors during DNA replication can lead to changes in nucleotide sequences, giving rise to new alleles.
- Recombination: As parental chromosomes exchange genetic material during meiosis, combinations of genes are generated, further increasing variation.
- Gene duplication: Occasional mistakes during replication can result in extra copies of genes, which may acquire different functions over time.
- Horizontal gene transfer: Bacteria and other microorganisms can transfer genetic material between individuals without direct offspring-parent relationships.
To appreciate the impact of these mechanisms on biodiversity and adaptation throughout history, let us examine Table 1 below:
Mechanism | Examples | Significance |
---|---|---|
Mutation | Point mutations | Can introduce novel traits or cause diseases |
Recombination | Crossing over during meiosis | Generates genetically unique offspring |
Gene duplication | Duplication of the CFTR gene | Allows for functional divergence and adaptation |
Horizontal transfer | Transfer of antibiotic resistance genes between bacteria | Facilitates rapid acquisition of advantageous traits in response to changing environments |
Table 1: Mechanisms of Genetic Variation
In conclusion, DNA replication is a vital process that ensures genetic stability during cell division and contributes to the diversity observed within populations. By understanding its mechanisms and consequences, scientists can gain insights into developmental disorders, as well as evolutionary processes shaping life on Earth.
References:
- Smith J, Doe A (Year). Title. Journal Name. Volume(Issue):Pages.
- Johnson B, et al. (Year). Title. Book Title. Publisher.