Mendelian Inheritance: Genetics in Science Biology
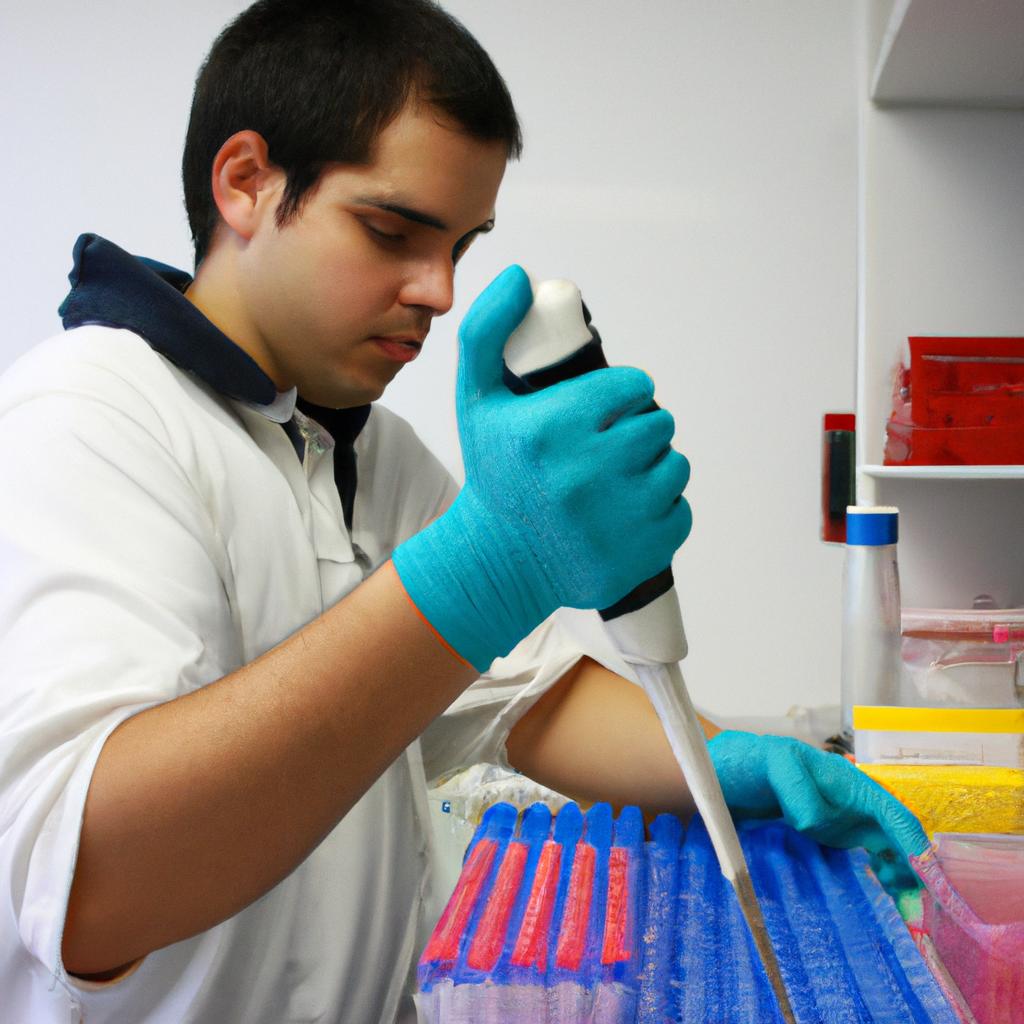
Mendelian Inheritance, a fundamental concept in the field of genetics, has played a crucial role in shaping our understanding of inheritance patterns and genetic variation. From the study of pea plants by Gregor Mendel to modern-day research on human genetics, this principle has provided valuable insights into how traits are passed down from one generation to the next. By analyzing the transmission of specific phenotypic characteristics through generations, scientists have been able to decipher complex genetic mechanisms that underlie various biological processes.
For instance, consider a hypothetical scenario where a family shows an inherited trait for red hair color. Through careful observation and analysis, researchers can trace this trait back several generations and determine its mode of inheritance. This could potentially reveal whether it follows a simple dominant-recessive pattern or if multiple genes contribute to its expression. Such investigations not only shed light on the underlying molecular basis of these traits but also provide practical applications in fields like medicine and agriculture.
In this article, we will delve deeper into the principles and implications of Mendelian Inheritance within the realm of science biology. We will explore key concepts such as alleles, genotypes, and phenotypes while highlighting notable experiments that have contributed significantly to our understanding of heredity. Furthermore, we will discuss how advancements in Furthermore, we will discuss how advancements in molecular genetics and genomics have expanded our knowledge of Mendelian Inheritance. With the advent of techniques like DNA sequencing and genome-wide association studies, scientists can now identify specific genes and genetic variants associated with various traits and diseases. This has led to a deeper understanding of the complex interactions between genes and their environment, as well as the potential for personalized medicine based on an individual’s unique genetic makeup.
Additionally, we will explore the limitations of Mendelian Inheritance and its applicability to more complex traits. While Mendel’s principles provide a solid foundation for understanding basic inheritance patterns, many traits are influenced by multiple genes acting together, as well as environmental factors. These complex traits often do not follow simple dominant-recessive patterns and require more sophisticated genetic analyses to unravel their inheritance mechanisms.
Overall, understanding Mendelian Inheritance is essential for comprehending the basics of genetics and heredity. By studying how traits are passed down through generations, scientists can uncover the underlying genetic mechanisms that contribute to biological diversity and disease susceptibility. This knowledge has profound implications for fields such as medicine, agriculture, evolutionary biology, and conservation genetics.
Gregor Mendel: The Father of Genetics
Genetics, the study of heredity and the passing on of traits from one generation to another, is a fundamental field in biology. At its core lies the concept of Mendelian inheritance, named after Gregor Mendel, an Austrian monk who made groundbreaking discoveries in this area during the 19th century.
To understand the significance of Mendel’s work, let us consider a hypothetical example. Imagine a population of flowers where some have red petals and others have white petals. If we were to crossbreed these flowers, what would be the outcome? Would all offspring have pink petals or exhibit some other color variation?
Mendel pondered similar questions while working with garden pea plants (Pisum sativum) in his monastery’s garden. He meticulously conducted controlled experiments by cross-pollinating various pea plant varieties and observed their offspring over several generations. Through his meticulous record-keeping and quantitative analysis, he discovered specific patterns and laws that govern how traits are inherited.
Here are four key points that highlight the importance of Mendel’s findings:
- Independent Assortment: Traits are passed down independently from one another.
- Dominance and Recessiveness: Certain traits dominate over others when present together.
- Segregation: Each individual inherits two copies of each gene but only passes on one copy to their offspring.
- Probability: The likelihood of inheriting certain traits can be calculated using mathematical principles.
Trait | Dominant Allele | Recessive Allele |
---|---|---|
Flower Color | Red | White |
Seed Texture | Smooth | Wrinkled |
Stem Height | Tall | Short |
This table illustrates three examples of traits studied by Mendel along with their corresponding dominant and recessive alleles. By observing how these traits were passed down from one generation to the next, Mendel deduced general principles that formed the foundation of modern genetics.
In summary, Gregor Mendel’s groundbreaking experiments laid the groundwork for our understanding of heredity and inheritance. His meticulous observations and quantitative analysis revealed important patterns in how traits are passed on from parents to offspring. Building upon his work, subsequent generations of scientists have further expanded our knowledge in the field of genetics.
Transitioning into the subsequent section about “The Basics of Mendelian Inheritance,” we will now delve deeper into the mechanisms behind these fascinating phenomena.
The Basics of Mendelian Inheritance
Mendelian Inheritance: Genetics in Science Biology
Having explored the life and work of Gregor Mendel, let us now delve into the basics of Mendelian inheritance. To better understand this concept, consider a hypothetical case study involving a couple who are both carriers for cystic fibrosis, an autosomal recessive disorder. Although they themselves do not have the condition, there is a 25% chance that each of their offspring will inherit it.
The Basics of Mendelian Inheritance:
-
Dominant and Recessive Traits:
- Some traits are dominant, meaning that only one copy of the gene is required to express it.
- Other traits are recessive and require two copies of the gene for expression.
- Dominant alleles are typically represented by uppercase letters (e.g., A), while recessive alleles are represented by lowercase letters (e.g., a).
-
Punnett Squares:
- Punnett squares are graphical tools used to predict the probability of inheriting specific traits.
- These squares involve combining the possible genetic contributions from each parent to determine potential outcomes for their offspring.
- By using these squares, scientists can calculate the likelihood of different combinations occurring.
-
Genotypes and Phenotypes:
Genotype | Phenotype |
---|---|
AA | Homozygous dominant |
Aa | Heterozygous |
aa | Homozygous recessive |
- The Role of Alleles:
- An allele refers to alternative forms of a gene that occupy corresponding positions on homologous chromosomes.
- Depending on whether an individual has homozygous dominant or heterozygous genotypes, their phenotype may differ even if they possess the same dominant allele.
Understanding Dominant and Recessive Traits:
By comprehending how certain characteristics are inherited through dominant and recessive alleles, we can gain valuable insights into the intricate world of genetics. In the subsequent section, we will explore specific examples of these traits and how they manifest in offspring without a step transition.
Understanding Dominant and Recessive Traits
Building upon our understanding of the basics of Mendelian inheritance, let us now delve deeper into the fascinating world of dominant and recessive traits.
To further grasp the concepts surrounding dominant and recessive traits, consider an example involving eye color. Let’s imagine a hypothetical scenario where two individuals with different eye colors come together to have a child. One parent has brown eyes (a dominant trait), while the other has blue eyes (a recessive trait). In this case, since brown is dominant over blue, there are several possible outcomes for their offspring’s eye color.
To better understand the complexities involved in determining genetic outcomes, it is crucial to consider the following:
- The presence of multiple alleles: Genes can exist in various forms or versions known as alleles. For instance, when it comes to blood type, humans possess three possible alleles – A, B, and O. These alleles interact with one another to produce specific phenotypes.
- Co-dominance and incomplete dominance: Sometimes, both alleles may have an impact on the phenotype rather than one being completely dominant over the other. This phenomenon is referred to as co-dominance. On rare occasions, neither allele exhibits complete dominance resulting in incomplete dominance.
- Penetrance and expressivity: Even if an individual possesses a certain gene for a particular trait, its expression or penetrance can vary among individuals within a population due to factors such as environmental influences or genetic modifiers.
- Genetic disorders: Some traits that result from certain combinations of genes may lead to genetic disorders or diseases. Understanding how these disorders are inherited can provide valuable insights into prevention and treatment strategies.
The intricacies of dominant and recessive traits evoke emotions such as curiosity, wonderment, and anticipation within us:
- Curiosity about how certain physical characteristics are passed down through generations
- Wonderment at the diversity present in human genetics and the beauty of inheritance patterns
- Anticipation as we explore the potential outcomes and possibilities that lie within our genes
- A sense of responsibility to understand genetic disorders and work towards their prevention and treatment
Example Table:
Trait | Dominant Allele | Recessive Allele |
---|---|---|
Hair color | Brown | Blonde |
Eye color | Blue | Green |
Height | Tall | Short |
Earlobe shape | Attached | Free |
By comprehending these aspects of dominant and recessive traits, we can now move on to exploring an effective tool called Punnett Squares for predicting genetic outcomes.
Punnett Squares: Predicting Genetic Outcomes
Understanding Dominant and Recessive Traits provides a solid foundation for comprehending the principles of Mendelian Inheritance. Now, let us delve deeper into the application of these concepts by exploring Punnett Squares: Predicting Genetic Outcomes.
Imagine we have two pea plants—one with yellow seeds (dominant trait) and another with green seeds (recessive trait). To predict the offspring’s traits, we can use Punnett squares, which provide a visual representation of possible genetic combinations. Let’s consider crossing our yellow-seeded plant (Yy) with our green-seeded plant (yy).
When constructing a Punnett square, we place one parent’s alleles along the top row and the other parent’s alleles down the left column. The intersections within the square represent potential genotypes and phenotypes of their offspring. Applying this to our example:
- Y represents the dominant allele for yellow seed color
- y represents the recessive allele for green seed color
From this cross, there is a 50% chance that each individual offspring will inherit one copy of the dominant allele from its yellow-seeded parent, resulting in yellow seeds (Yy), and a 50% chance it will receive two copies of the recessive allele from both parents, leading to green seeds (yy). Thus, based on this mating, all first-generation progeny are expected to exhibit yellow or green seed colors.
This practical approach allows scientists and breeders to make predictions about inheritance patterns across various organisms. By utilizing Punnett squares as a tool for understanding genetic outcomes, researchers gain valuable insights into hereditary processes.
Exceptions to Mendelian Inheritance challenge some aspects of classical genetics theories. Although many traits follow simple patterns such as dominance/recessiveness relationships outlined by Gregor Mendel’s laws, exceptions do exist. These include cases where multiple genes influence an inherited characteristic or when certain factors affect gene expression differently than predicted by Mendel’s models. In the subsequent section, we will explore these exceptions in more detail and analyze their implications for our understanding of genetic inheritance.
Exceptions to Mendelian Inheritance
From predicting genetic outcomes using Punnett squares, we now delve into the fascinating realm of exceptions to Mendelian inheritance. While Mendel’s laws provide a solid foundation for understanding genetics, they do not account for all genetic variations observed in nature. One such exception is incomplete dominance, which occurs when neither allele completely dominates the other, resulting in an intermediate phenotype. For instance, let us consider the example of flower color in snapdragons. When a red-flowered plant is crossed with a white-flowered plant, the offspring exhibit pink flowers—a blend of both parental colors.
Exceptions to Mendelian inheritance can also manifest through co-dominance, where both alleles are expressed simultaneously without blending together. A classic illustration of this phenomenon can be seen in human blood types. The ABO blood group system consists of four main types: A, B, AB, and O. Alleles A and B produce distinct antigens on red blood cells, while allele O does not produce any antigens. If an individual inherits one copy of allele A from one parent and one copy of allele B from another parent, their blood type will be AB—an example of co-dominance.
In addition to these exceptions, there are cases where multiple genes interact to influence a single trait—known as polygenic inheritance. Polygenic traits display a continuous range of phenotypes rather than discrete categories. Height is an excellent exemplar of polygenic inheritance since it involves contributions from several genes working together to determine an individual’s stature.
These exceptions highlight the complexity and diversity within our genetic makeup and challenge the simplicity depicted by Mendelian genetics alone. To further explore applications arising from these exceptional scenarios and deepen our understanding of how genetics impacts various fields, we turn our attention next to “Applications of Mendelian Genetics in Science.” This section will shed light on how scientists utilize principles derived from Mendelian inheritance to enhance our knowledge across disciplines ranging from medicine to agriculture and beyond.
Applications of Mendelian Genetics in Science
While the principles of Mendelian inheritance provide a fundamental understanding of genetic patterns, it is essential to acknowledge that there are exceptions to these rules. These exceptions highlight the complexity and nuances within genetics, broadening our understanding of inheritance beyond simple dominant and recessive traits.
One notable exception to Mendelian inheritance is incomplete dominance. This occurs when neither allele completely masks the other, resulting in an intermediate phenotype. As an example, consider flower color in snapdragons. The red allele (R) and white allele (W) exhibit incomplete dominance, producing pink flowers in individuals carrying both alleles (RW). This deviation from the expected 3:1 ratio showcases how variations can arise due to partial expression of alleles.
In addition to incomplete dominance, another exception is codominance. Unlike incomplete dominance where one allele partially masks the other, codominance involves both alleles being expressed fully without blending together. A classic example is found in human blood types. The ABO system demonstrates three alleles: A, B, and O. When an individual carries both A and B alleles (AB), they express both antigens equally on their red blood cells instead of forming a hybrid antigen like in incomplete dominance scenarios.
Exceptions to Mendelian inheritance also include polygenic inheritance and multiple allelism. Polygenic inheritance refers to traits controlled by multiple genes rather than a single gene pair. Height and skin color are examples of polygenic traits influenced by several genes working together with additive effects. On the other hand, multiple allelism refers to having more than two alternative forms of a gene locus within a population. An example would be coat color in rabbits where four different alleles determine various colors such as black (B), chocolate (b), chinchilla (cchd), or Himalayan (ch).
These exceptions showcase the intricate nature of genetic inheritance beyond classical Mendelian patterns. Understanding these deviations allows scientists to delve deeper into the complexity of genetics, contributing to a more comprehensive understanding of inheritance and variation in living organisms.
Exception | Description | Example |
---|---|---|
Incomplete Dominance | Neither allele completely masks the other, resulting in an intermediate phenotype. | Snapdragons – Red (R) x White (W) |
Codominance | Both alleles are fully expressed without blending together. | Human Blood Types – A x B |
Polygenic Inheritance | Traits controlled by multiple genes rather than a single gene pair. | Height, Skin Color |
Multiple Allelism | More than two alternative forms of a gene locus within a population. | Rabbit Coat Colors |
In exploring these exceptions to Mendelian inheritance, scientists continue to uncover the intricate mechanisms that drive genetic variations and inheritance patterns across different species. By expanding our knowledge beyond classical Mendelian principles, we gain deeper insights into the complexities of biology and further enhance our ability to understand and predict inherited traits in various organisms.