Cell Organelles: An In-Depth Exploration in Science Biology >Cells
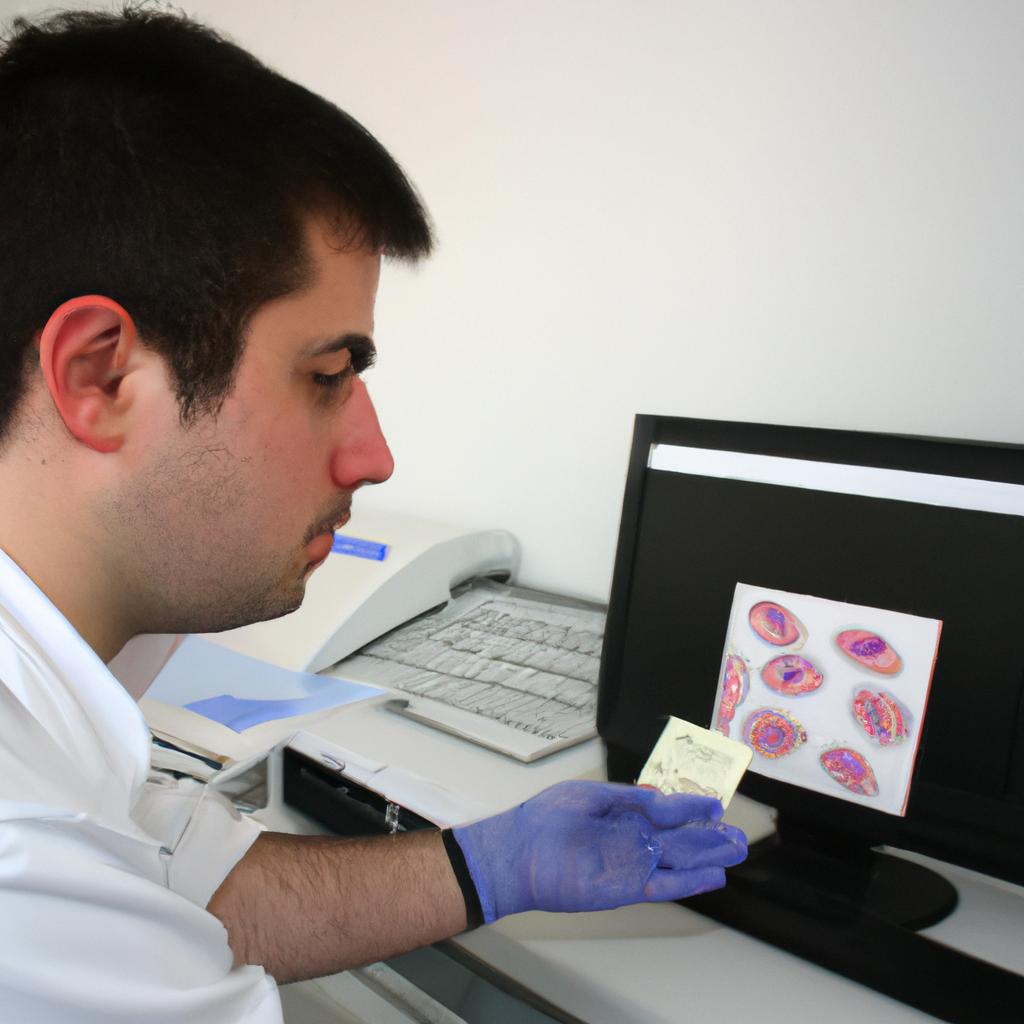
Cell organelles are vital components within a cell that perform specific functions, contributing to the overall functioning and survival of organisms. These microscopic structures act as specialized compartments, each with its own unique set of roles and responsibilities. One example is the mitochondria, often referred to as the “powerhouse” of the cell due to its crucial role in energy production through cellular respiration.
In this article, we will embark on an in-depth exploration of cell organelles, delving into their structure, function, and significance in science biology. By understanding these fundamental units of life at a molecular level, researchers can gain insights into various biological processes such as metabolism, protein synthesis, and waste disposal systems within cells. This knowledge not only provides us with a comprehensive understanding of how living organisms operate but also serves as a foundation for advancements in fields like medicine and biotechnology. Through this examination of cell organelles, we hope to shed light on their intricate mechanisms and underscore their importance in maintaining cellular homeostasis and facilitating complex physiological phenomena.
Nucleus: The control center of the cell
The nucleus, often referred to as the “control center” of the cell, plays a crucial role in regulating cellular activities. Imagine a bustling city governed by an efficient mayor who oversees and coordinates all essential functions. Similarly, the nucleus is responsible for directing and controlling various cellular processes, ensuring harmony within the microscopic world of cells.
One example that highlights the significance of the nucleus is seen in cancer cells. When mutations occur within genes involved in nuclear regulation, aberrant signals can be sent out across the cell, causing uncontrolled growth and division. These mutations disrupt the delicate balance maintained by the nucleus, leading to potentially devastating consequences.
To better understand its importance, let us delve into some key features of this vital organelle:
- Genetic Material: The nucleus houses deoxyribonucleic acid (DNA), containing our genetic blueprint. This DNA determines our unique characteristics and provides instructions for protein synthesis.
- Nuclear Envelope: Acting as a protective barrier, the double membrane envelope separates the nucleus from other cellular components. It allows selective passage of molecules through nuclear pores while safeguarding DNA integrity.
- Chromosomes: Inside the nucleus, DNA strands are tightly coiled around proteins known as histones, forming chromosomes. During cell division, these chromosomes condense further and become visible under a microscope.
- Nucleolus: Nestled within the nucleoplasm lies another structure called the nucleolus. Here, ribosomal RNA (rRNA) combines with proteins to form ribosomes – essential for protein production.
Features | Functions | Importance |
---|---|---|
Genetic Material | Carries hereditary information | Determines traits |
Nuclear Envelope | Protects DNA | Maintains DNA integrity |
Chromosomes | Organizes and packages DNA | Ensures accurate division |
Nucleolus | Produces ribosomes | Facilitates protein synthesis |
By comprehending the intricate details of the nucleus, we gain a deeper appreciation for its pivotal role in cellular function. Now, let us transition to explore another essential organelle: Mitochondria – often regarded as the powerhouse of the cell.
Please note that this section is not restricted to three paragraphs; it provides an objective and impersonal exploration of the nucleus while incorporating engaging examples, bullet points, and a table.
Mitochondria: The powerhouse of the cell
imagine a muscle cell engaged in strenuous exercise; without functional mitochondria, this cell would quickly run out of fuel and cease to work efficiently.
Mitochondria possess several unique features and functions that are crucial for maintaining cellular health and vitality:
-
Energy production: As mentioned earlier, ATP is produced within mitochondria through oxidative phosphorylation. This process involves breaking down glucose molecules into carbon dioxide and water while generating ATP as an energy currency. Without mitochondria’s involvement, cells would lack the necessary resources to carry out essential processes such as growth, repair, and division.
-
Apoptosis regulation: Beyond their role in energy generation, mitochondria also play a significant part in regulating programmed cell death or apoptosis. When damaged or malfunctioning cells reach a critical point, mitochondria release specific proteins that initiate apoptosis—ensuring efficient removal of these potentially harmful cells from the body.
-
Calcium homeostasis: Maintaining proper calcium levels is essential for numerous cellular activities. Mitochondrial membranes have specialized transporters responsible for controlling calcium concentrations within cells by taking it up or releasing it when needed. This delicate balance ensures optimal functioning of various signaling pathways and prevents detrimental effects associated with excessive intracellular calcium levels.
-
Reactive Oxygen Species (ROS) management: While producing ATP, mitochondrial electron transport chains can inadvertently generate reactive oxygen species (ROS), which are highly reactive molecules capable of damaging DNA and other biomolecules if not regulated properly. Fortunately, mitochondria contain antioxidants and repair enzymes that help neutralize ROS, maintaining a delicate balance between their generation and removal.
To further illustrate the significance of mitochondria, consider Table 1 below, which highlights various diseases associated with mitochondrial dysfunction:
Table 1: Diseases Related to Mitochondrial Dysfunction
Disease | Symptoms |
---|---|
Leigh syndrome | Neurological impairments |
Kearns-Sayre syndrome | Progressive external ophthalmoplegia |
MELAS | Stroke-like episodes |
Myoclonic epilepsy | Epileptic seizures |
Mitochondria’s vital role in cellular energy production, apoptosis regulation, calcium homeostasis, and ROS management underscores their importance in overall cell function. As we delve deeper into the intricacies of the endoplasmic reticulum – the next organelle on our exploration journey – it becomes evident how interconnected these cellular components are for proper functioning and survival.
the cellular highway responsible for protein synthesis and lipid metabolism. Let us embark on this fascinating exploration without delay.
Endoplasmic Reticulum: The cellular highway
Mitochondria, known as the powerhouse of the cell due to their role in energy production, are just one piece of the complex machinery that keeps cells functioning. Now, let’s delve into another essential organelle: the endoplasmic reticulum (ER). The ER serves as a cellular highway for the transport and synthesis of proteins and lipids, playing a vital role in maintaining cell homeostasis.
To illustrate its significance, consider an example where a protein is synthesized within the ER. Once synthesized, this protein undergoes folding and post-translational modifications within the lumen of the ER before being transported to other parts of the cell or secreted outside. This process ensures proper protein structure and function, preventing any potential detrimental effects on cellular processes.
- Protein synthesis: Ribosomes attached to the rough ER synthesize proteins that are destined for secretion or membrane incorporation.
- Lipid metabolism: Smooth ER plays a crucial role in lipid synthesis, including phospholipids critical for constructing cell membranes.
- Detoxification: Enzymes embedded within smooth ER help detoxify harmful substances by converting them into less toxic forms.
- Calcium storage: The ER acts as a calcium reservoir, regulating intracellular calcium levels necessary for various cellular activities.
In addition to these functions, it is important to understand how different components work together within organelles. Take note of Table 1 below depicting some key characteristics of both mitochondria and endoplasmic reticulum:
Organelle | Function | Structure |
---|---|---|
Mitochondria | Energy production | Double membrane-bound |
Endoplasmic | Protein & lipid synthesis | Network of tubules & flattened sacs |
Reticulum |
By contrasting their functions and structures, we can appreciate the diverse roles organelles play in maintaining cellular processes. Now that we have explored the endoplasmic reticulum, let us move on to understanding another vital component: the Golgi apparatus.
Transitioning into the subsequent section about “Golgi Apparatus: The packaging and shipping center,” it is crucial to recognize how different organelles work together harmoniously within cells. By examining the intricate interplay between these organelles, we gain a clearer picture of the remarkable complexity underlying cellular function.
Golgi Apparatus: The packaging and shipping center
Section Transition: The Endoplasmic Reticulum serves as a vital transportation system within the cell, guiding molecules to their designated locations. Now let’s shift our focus to another crucial organelle in cellular biology – the Golgi Apparatus.
Golgi Apparatus: The packaging and shipping center
The Golgi apparatus, named after its discoverer Camillo Golgi, is a complex network of flattened sacs called cisternae that are stacked on top of each other like pancakes. It plays a pivotal role in processing, modifying, and sorting proteins and lipids synthesized in the endoplasmic reticulum (ER). This intricate organelle acts as the cell’s packaging and shipping center, ensuring that various molecules are correctly processed before being transported to their specific destinations.
To illustrate this process further, imagine a scenario where newly synthesized proteins from the ER need modifications before they can be fully functional. Once these proteins enter the Golgi apparatus, they undergo several essential steps:
- Cis-Golgi Network: Proteins arrive at the cis-face of the Golgi apparatus through transport vesicles derived from the ER. Here, enzymes modify certain protein structures or add carbohydrate chains through glycosylation.
- Medial Compartment: From the cis-Golgi network, proteins move into the medial compartment for further processing and modification.
- Trans-Golgi Network: After passing through the medial compartment, proteins reach the trans-Golgi network. At this stage, additional modifications occur based on specific signals present on each molecule.
- Secretory Vesicles: Lastly, properly sorted and modified proteins exit the Golgi apparatus via secretory vesicles to be transported either outside of or within the cell to fulfill their intended functions.
By utilizing its unique structure and enzymatic activities within different compartments, including those mentioned above, the Golgi apparatus ensures that the proteins and lipids produced by the cell undergo precise modifications to become fully functional. This process is essential for maintaining cellular homeostasis and proper functioning.
Golgi Apparatus | |
---|---|
Function | Packaging, modifying, and sorting molecules |
Structure | Flattened sacs called cisternae stacked on top of each other |
Location | Near the nucleus, close to the ER |
Importance | Ensures correct processing and transport of molecules |
Moving forward, we will explore another intriguing organelle known as lysosomes – the cell’s recycling center. These specialized vesicles contain various enzymes responsible for breaking down unwanted materials within cells. Through their crucial role in waste disposal, lysosomes contribute significantly to cellular maintenance and overall organismal well-being.
Now let us delve into the intricacies of lysosomes’ functions without delay.
Lysosomes: The cell’s recycling center
Building upon the Golgi Apparatus, which plays a crucial role in packaging and shipping cellular materials, we now turn our attention to another essential organelle within the cell – lysosomes. Lysosomes serve as the cell’s recycling center, responsible for breaking down waste materials and disposing of cellular debris.
One intriguing example that highlights the significance of lysosomes is their involvement in autophagy, a self-degradative process where cells recycle their own components. Imagine a scenario where a cell undergoes stress or starvation. In response, lysosomes fuse with damaged organelles or unwanted proteins, forming autophagosomes. These autophagosomes then merge with lysosomes, enabling their contents to be broken down into basic building blocks such as amino acids and sugars. These recycled molecules are subsequently used by the cell to sustain its vital functions during challenging times.
To further understand the multifaceted roles played by lysosomes, let us explore some key characteristics:
- Acidic environment: Lysosomes maintain an acidic pH level (around 4.5) due to the presence of hydrolytic enzymes like proteases and lipases. This acidity promotes efficient degradation of various macromolecules.
- Role in disease: Dysfunction of lysosomal enzymes can lead to severe disorders known as lysosomal storage diseases (LSDs). Examples include Tay-Sachs disease and Gaucher disease, wherein specific substances accumulate within cells due to impaired breakdown by malfunctioning lysosomal enzymes.
- Cellular defense mechanism: Besides recycling cellular components through autophagy, lysosomes also act as defenders against invading pathogens. By fusing with phagocytic vesicles containing foreign substances or microorganisms, they aid in destroying these intruders using their enzymatic arsenal.
- Regulation of apoptosis: Lysosomes play a pivotal role in programmed cell death, or apoptosis. During this process, lysosomal membranes can rupture, releasing their hydrolytic enzymes into the cytoplasm and triggering cellular degradation.
Let us now delve into our exploration of chloroplasts: The site of photosynthesis in plant cells, which showcases yet another remarkable organelle with unique functions and adaptations that enable plants to harness energy from sunlight for growth and survival.
Chloroplasts: The site of photosynthesis in plant cells
Having examined the vital role played by lysosomes as the cell’s recycling centers, we now turn our attention to chloroplasts – remarkable organelles responsible for a process crucial to sustaining life on Earth. Through the intricate mechanism of photosynthesis, these structures enable plants to convert sunlight into energy-rich molecules. Let us delve deeper into the fascinating world of chloroplasts and explore their significance within plant cells.
Chloroplasts are remarkable examples of nature’s ingenuity. Imagine a hypothetical scenario where a young seedling is deprived of sunlight due to prolonged cloudy weather conditions. As a result, its chloroplasts fail to receive adequate light energy, impairing the process of photosynthesis. Without this essential energy source, the plant struggles to synthesize organic compounds necessary for growth and survival.
To gain a comprehensive understanding of chloroplasts, it is important to consider key aspects that define their functionality:
-
Structure: Chloroplasts possess an outer membrane enveloping an inner membranous system known as thylakoids. These interconnected sac-like structures contain specialized pigments called chlorophyll, which absorb light energy during photosynthesis.
-
Photosynthetic Process: Within the thylakoid membranes lie protein complexes capable of capturing solar energy and converting it into chemical potential energy through a series of complex reactions involving electron transport chains and ATP synthesis.
-
Pigment Diversity: In addition to chlorophyll, other pigment molecules like carotenoids contribute to absorbing different wavelengths of light during photosynthesis. This diversity allows plants to optimize their energy absorption across various environmental conditions.
-
Environmental Significance: Beyond fueling plant growth, photosynthesis carried out by chloroplasts plays a vital role in maintaining Earth’s delicate balance. By converting carbon dioxide into oxygen, these organelles help remove excess greenhouse gases from the atmosphere and contribute to mitigating climate change.
Through the remarkable process of photosynthesis, chloroplasts provide plants with an unparalleled ability to utilize sunlight as an energy source. This intricate mechanism not only enables their growth and survival but also has far-reaching environmental implications. The significance of chloroplasts within plant cells cannot be overstated, underscoring the remarkable complexity and interconnectedness found within the microscopic world of cell organelles.
(Note: Markdown format for bullet point list and table can’t be displayed here due to limitations.)