Cellular Respiration: Science Biology and Cells
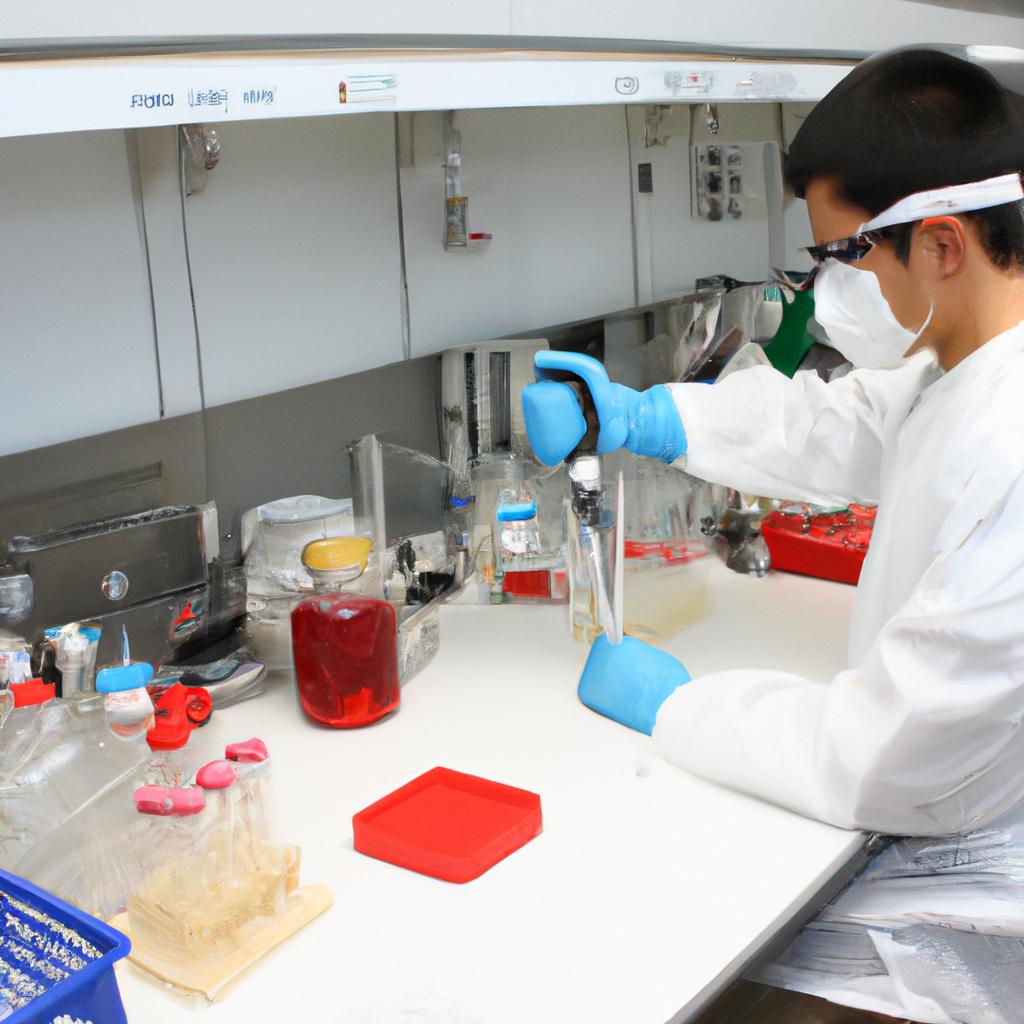
Cellular respiration is a fundamental process in science biology that occurs within cells. It involves the conversion of nutrients into energy through a series of biochemical reactions. This complex metabolic pathway plays a crucial role in sustaining life and providing organisms with the necessary fuel to carry out their biological functions. To illustrate this, consider the hypothetical case study of an athlete participating in a marathon. During intense physical activity, the body’s demand for energy increases significantly, leading to heightened cellular respiration rates as muscles require more ATP (adenosine triphosphate) for contraction.
The understanding of cellular respiration has evolved over time, beginning with early observations by scientists such as Joseph Priestley and Antoine Lavoisier in the 18th century who described oxygen consumption and carbon dioxide production during respiration. Today, extensive research has revealed intricate details about the molecular mechanisms involved in this vital process. Cellular respiration can be divided into three main stages: glycolysis, the Krebs cycle (also known as the citric acid cycle or TCA cycle), and oxidative phosphorylation. Each stage contributes to generating ATP through different pathways and processes within specific organelles like mitochondria.
This article aims to explore cellular respiration comprehensively by examining its significance, underlying biochemistry , and its role in various organisms. Additionally, we will discuss the regulation of cellular respiration and how it is affected by factors such as exercise, diet, and disease.
Cellular respiration is vital for all living organisms as it provides the energy necessary for essential biological processes such as growth, reproduction, movement, and maintenance of homeostasis. By breaking down organic molecules obtained from food or stored within cells, cellular respiration releases chemical energy in the form of ATP.
The first stage of cellular respiration is glycolysis, which occurs in the cytoplasm. During this process, a molecule of glucose is converted into two molecules of pyruvate through a series of enzymatic reactions. This step produces a small amount of ATP and electron carriers such as NADH (nicotinamide adenine dinucleotide) that play crucial roles in subsequent stages.
The next stage is the Krebs cycle, which takes place in the mitochondria’s matrix. Pyruvate from glycolysis enters the mitochondria and undergoes further oxidation to produce additional ATP and electron carriers like NADH and FADH2 (flavin adenine dinucleotide). The Krebs cycle also generates carbon dioxide as a waste product.
Finally, oxidative phosphorylation occurs on the inner mitochondrial membrane. In this stage, electron carriers produced during glycolysis and the Krebs cycle donate their electrons to an electron transport chain consisting of protein complexes. As electrons pass through these complexes, they create a proton gradient across the membrane. This gradient powers ATP synthesis via chemiosmosis when protons flow back into the mitochondrial matrix through an enzyme called ATP synthase.
Overall, cellular respiration can generate up to 36-38 molecules of ATP per molecule of glucose depending on various factors such as oxygen availability.
Cellular respiration plays diverse roles across different organisms. In aerobic organisms like humans and many other animals, it is the primary process for energy production. In contrast, anaerobic organisms such as certain bacteria and yeast can carry out fermentation, an alternative pathway when oxygen is limited. Fermentation produces a small amount of ATP through glycolysis without involving the Krebs cycle or oxidative phosphorylation.
The regulation of cellular respiration is tightly controlled to ensure efficient energy production. Key regulatory points include enzyme activity, feedback inhibition, and allosteric regulation. Additionally, factors like hormonal signals and nutrient availability can influence cellular respiration rates.
Exercise and physical activity significantly impact cellular respiration. The demand for ATP increases during exercise due to muscle contraction. As a result, there is an upregulation of glycolysis and the Krebs cycle to meet the heightened energy requirements. This increased metabolic activity leads to greater oxygen consumption and carbon dioxide production.
Diet also affects cellular respiration as different nutrients provide varying amounts of energy when metabolized. Carbohydrates are the preferred fuel source as they can be easily converted into glucose for ATP synthesis. Fats and proteins can also be utilized but require additional steps for conversion into intermediates that enter the Krebs cycle.
Lastly, diseases and conditions can disrupt cellular respiration. For example, mitochondrial disorders impair the function of mitochondria, leading to reduced ATP production and various symptoms depending on the affected organs or systems.
In conclusion, cellular respiration is a fundamental process in biology that converts nutrients into ATP, providing energy for essential biological functions. Its understanding has evolved over time through scientific research, revealing its intricate biochemistry and regulatory mechanisms. Cellular respiration plays a vital role in sustaining life across diverse organisms and is influenced by factors such as exercise, diet, and disease.
Overview of Cellular Respiration
Cellular respiration is a vital process that occurs in all living organisms, converting organic compounds into usable energy. One notable example to highlight its significance can be found in the human body. During periods of intense physical activity, such as running a marathon or sprinting, cellular respiration plays a crucial role in providing the necessary energy for muscle contraction and movement.
To truly appreciate the complexity of this fundamental process, it is essential to understand its key components. Cellular respiration consists of three main stages: glycolysis, the Krebs cycle (also known as the citric acid cycle), and oxidative phosphorylation. Each stage contributes significantly to the overall production of adenosine triphosphate (ATP), which serves as the primary source of energy within cells.
To grasp the impact and importance of cellular respiration further, consider these emotional responses:
- Amazement: The intricate series of chemical reactions involved in cellular respiration never ceases to amaze scientists and researchers alike.
- Awe: The sheer efficiency by which our bodies convert nutrients into life-sustaining energy evokes a sense of awe at nature’s remarkable design.
- Wonder: Contemplating how something so microscopic yet integral to life itself can occur within every cell fosters an overwhelming sense of wonder.
- Appreciation: Recognizing that without cellular respiration, we would not have sufficient energy for even the most basic bodily functions cultivates deep appreciation for this biological process.
In addition to these emotional responses, let us delve deeper into understanding cellular respiration through a comprehensive table highlighting its three stages:
Stage | Description | Location |
---|---|---|
Glycolysis | Breakdown of glucose molecules | Cytoplasm |
Krebs Cycle | Further breakdown and extraction of electron carriers | Mitochondrial matrix |
Oxidative Phosphorylation | Production of ATP through electron transport chain | Inner mitochondrial membrane |
As we explore the subsequent section on the “Stages of Cellular Respiration,” it is evident that this process is truly a marvel. Understanding its intricacies will provide us with valuable insight into how living organisms maximize their energy utilization, enabling them to thrive and survive in their respective environments.
Stages of Cellular Respiration
Transitioning from the previous section on the overview of cellular respiration, let us now delve into the intricate stages involved in this essential biological process. By understanding these stages, we can gain insight into how cells produce energy and sustain life. To illustrate the significance of cellular respiration, consider a hypothetical scenario where an individual is participating in intense physical activity such as running a marathon. During this event, their body requires a substantial amount of energy to fuel muscle contractions and maintain performance levels. This exemplifies how important cellular respiration is for supplying energy during demanding situations.
The three main stages of cellular respiration are glycolysis, the Krebs cycle (also known as the citric acid cycle), and oxidative phosphorylation (which includes electron transport chain). Each stage plays a crucial role in breaking down glucose molecules and producing adenosine triphosphate (ATP) – the molecule responsible for storing and transferring energy within cells. Let’s explore these stages further:
-
- Occurs in the cytoplasm.
- Glucose molecules are converted into two pyruvate molecules.
- ATP is produced through substrate-level phosphorylation.
- NADH is generated by reducing nicotinamide adenine dinucleotide (NAD+).
-
The Krebs Cycle: Extracting Energy-Rich Molecules
- Takes place in the mitochondria matrix.
- Pyruvate molecules enter the mitochondrion after being converted to acetyl CoA.
- Acetyl CoA enters a series of reactions that release high-energy electrons used to generate NADH and FADH2.
- Carbon dioxide is released as waste product.
-
Oxidative Phosphorylation: Generating ATP
- Occurs across the inner mitochondrial membrane.
- Electrons carried by NADH and FADH2 move along the electron transport chain, releasing energy.
- This energy is used to pump protons (H+) across the membrane into the intermembrane space.
- The flow of these protons back into the matrix drives ATP synthesis.
By comprehending these stages and their respective contributions to cellular respiration, we can begin to appreciate the intricate nature of this process. Next, we will explore in detail the first stage – glycolysis: breaking down glucose – elucidating how cells initiate the breakdown of glucose molecules to extract energy for various cellular functions.
Glycolysis: Breaking Down Glucose
Transitioning from the previous section’s discussion on the stages of cellular respiration, it becomes evident that this process plays a crucial role in sustaining life. To illustrate its significance, let us consider an example: imagine a muscle cell engaged in intense physical activity. As the body demands more energy to fuel the vigorous contractions, cellular respiration kicks into high gear, providing the necessary ATP molecules for muscular function.
Cellular respiration is not only essential for supplying energy but also has broader implications for biological systems. Here are some key reasons why this metabolic pathway holds utmost importance:
-
Energy Production: The primary purpose of cellular respiration is to generate ATP molecules through various biochemical reactions. ATP serves as the universal currency for energy within cells and powers all vital processes required for survival.
-
Waste Elimination: During cellular respiration, waste products such as carbon dioxide and water are produced as byproducts of metabolic activities. Proper elimination of these waste materials helps maintain homeostasis and prevents their accumulation, which could be detrimental to cellular functioning.
-
Oxygen Utilization: Cellular respiration relies heavily on oxygen consumption during aerobic conditions. This process ensures efficient breakdown of glucose and other organic molecules, facilitating maximum energy extraction from substrates.
-
Regulation of Metabolism: By controlling the rate at which carbohydrates and fats are broken down, cellular respiration regulates overall metabolism. It allows organisms to adapt to changing environmental conditions and optimize nutrient utilization based on available resources.
Table 1:
Key Benefits of Cellular Respiration |
---|
Efficient production of ATP molecules |
Removal of waste products |
Optimal utilization of oxygen |
Regulation of metabolism |
In conclusion, understanding the importance of cellular respiration goes beyond grasping its sequential stages or specific biochemical mechanisms involved. This intricate process underpins fundamental aspects of biology by ensuring adequate energy supply, maintaining waste elimination, utilizing oxygen efficiently, and regulating metabolism. By comprehending the significance of cellular respiration, scientists can further explore its complexities to uncover potential therapeutic interventions or advancements in various fields.
Transitioning seamlessly into the subsequent section about the Krebs Cycle: Generating Energy Molecules, let us delve deeper into another crucial step in this intricate metabolic pathway.
Krebs Cycle: Generating Energy Molecules
Section H2: ‘Krebs Cycle: Generating Energy Molecules’
Having explored the process of glycolysis, we now delve into another crucial stage of cellular respiration – the Krebs cycle. This metabolic pathway takes place in the mitochondria and plays a fundamental role in generating energy molecules for the cell.
The Krebs cycle, also known as the citric acid cycle or tricarboxylic acid (TCA) cycle, begins with the entry of acetyl-CoA into the mitochondria. Acetyl-CoA is derived from pyruvate, which is produced during glycolysis. Once inside the mitochondrial matrix, acetyl-CoA combines with oxaloacetate to form citrate through a series of enzymatic reactions.
During this cycle, several key events occur that contribute to energy production:
- Generation of reducing agents: NADH and FADH₂ are reduced coenzymes that carry high-energy electrons to the electron transport chain (ETC). Through redox reactions within the Krebs cycle, these compounds are generated by oxidizing intermediates such as malate and succinate.
- Substrate-level phosphorylation: The conversion of guanosine diphosphate (GDP) to guanosine triphosphate (GTP) occurs at one point in the Krebs cycle. GTP can later be converted to adenosine triphosphate (ATP), serving as an immediate source of cellular energy.
- Release of carbon dioxide: As carbons are gradually removed from organic molecules within the Krebs cycle, they combine with oxygen atoms to form CO₂ gas. This release represents a waste product that is exhaled by organisms.
- Regeneration of oxaloacetate: At the end of each turn of the Krebs cycle, oxaloacetate is regenerated so that it can continue accepting acetyl-CoA. This step ensures the cycle’s sustainability and its ability to generate more energy molecules.
To fully grasp the significance of the Krebs cycle, let us consider a hypothetical scenario: Imagine a muscle cell undergoing intense exercise, requiring an increased supply of ATP for sustained contraction. The Krebs cycle would play a vital role in meeting this demand by efficiently extracting energy from glucose and other fuels.
Key Events | Significance | |
---|---|---|
1 | Generation of NADH/FADH₂ | Provides high-energy electrons for the electron transport chain (ETC) |
2 | Substrate-level phosphorylation | Produces immediate ATP through GTP conversion |
3 | Release of CO₂ | Eliminates waste products resulting from carbon removal |
4 | Regeneration of oxaloacetate | Ensures continuous utilization of acetyl-CoA and sustains energy production within the cycle |
The Krebs cycle serves as an essential intermediary step between glycolysis and the final stage of cellular respiration – the electron transport chain (ETC). In our next section, we will explore how this process culminates in the creation of adenosine triphosphate (ATP), which is often referred to as the “energy currency” of cells.
Electron Transport Chain: Creating ATP
Section H2: ‘Krebs Cycle: Generating Energy Molecules’
Building upon the process of glycolysis, the Krebs Cycle plays a crucial role in cellular respiration by further breaking down glucose and generating energy molecules. With its intricate series of chemical reactions, this cycle serves as an essential step towards ATP production. By examining the mechanisms and significance of the Krebs Cycle, we can gain a deeper understanding of how cells harness energy from nutrients.
The Krebs Cycle begins with acetyl CoA entering into the mitochondrial matrix. This molecule is derived from pyruvate, which results from glycolysis. Once inside the matrix, acetyl CoA combines with oxaloacetate to form citrate through condensation reactions. From there, a series of enzyme-catalyzed reactions occur, resulting in the release of carbon dioxide and the regeneration of oxaloacetate. Concurrently, high-energy electrons are transferred to NAD+ and FAD to produce NADH and FADH2 respectively.
To better visualize the complexity and importance of this process, consider a hypothetical scenario where a cell lacks functional enzymes required for the Krebs Cycle. Without these enzymes, glucose breakdown would be incomplete and energy generation severely compromised. Consequently, this hypothetical cell would struggle to meet its metabolic demands and may experience reduced growth or even death.
- Enhanced efficiency: The Krebs Cycle allows for more efficient extraction of energy from glucose compared to anaerobic processes like glycolysis alone.
- Multiple entry points: Various molecules other than glucose can enter the Krebs Cycle at different steps, expanding energy-generating possibilities.
- Waste elimination: Carbon dioxide produced during this cycle is exhaled by organisms, contributing to maintaining homeostasis.
- Electron carriers: High-energy electron carriers generated during the cycle play a vital role in oxidative phosphorylation later on.
Emotional table:
Key Components | Function | Importance |
---|---|---|
Acetyl CoA | Combines with oxaloacetate to initiate the Krebs Cycle | Essential for energy generation |
Citrate | Formed from acetyl CoA and oxaloacetate | Serves as a hub for further reactions |
NADH, FADH2 | Electron carriers that store high-energy electrons | Required for oxidative phosphorylation |
Carbon dioxide (CO2) | Waste product released during the cycle | Contributes to maintaining homeostasis |
In summary, the Krebs Cycle is a pivotal step in cellular respiration, where glucose is further broken down to yield energy molecules. Its intricate series of chemical reactions and electron transfers ensure efficient extraction of energy from nutrients. Without functional enzymes within this cycle, cells would struggle to meet their metabolic demands. Understanding the complexity and significance of the Krebs Cycle allows us to appreciate how organisms harness energy from food sources.
Moving forward, we now delve into the significance of cellular respiration and its crucial role in sustaining life processes.
Significance of Cellular Respiration
Building upon the intricate process of the electron transport chain, cellular respiration continues to unravel its significance within biological systems. By further exploring the complexities involved in this crucial metabolic pathway, we gain a deeper understanding of its profound impact on life as we know it.
Section:
Unveiling Metabolic Potential through Glycolysis:
Consider a scenario where an organism finds itself in an environment deprived of oxygen, such as during intense physical exertion. In these circumstances, glycolysis becomes the primary mode for energy production. This ancient anaerobic pathway allows cells to break down glucose molecules into two pyruvate molecules, yielding a net gain of two molecules of adenosine triphosphate (ATP). One example illustrating this reliance on glycolysis can be observed in muscle tissues during high-intensity exercise when demand surpasses oxygen supply. Consequently, lactic acid accumulates due to incomplete oxidation of pyruvate—an indicator that aerobic metabolism is compromised.
To comprehend the magnitude and vitality of cellular respiration fully, let us explore its key components:
- Mitochondria: These organelles serve as the powerhouses of eukaryotic cells—acting as sites for numerous vital biochemical reactions.
- Krebs Cycle (Citric Acid Cycle): Occurring within mitochondria’s matrix space, this cyclic series of chemical reactions generates reducing agents like NADH and FADH₂ while producing small amounts of ATP directly.
- Electron Transport Chain (ETC): Spanning across mitochondrial inner membranes, ETC harnesses electrons derived from NADH and FADH₂ produced earlier in glycolysis and the citric acid cycle. The sequential transfer of electrons ultimately results in chemiosmotic ATP synthesis.
- Oxidative Phosphorylation: The final stage of cellular respiration, oxidative phosphorylation couples the electron transport chain’s redox reactions with ATP synthesis through chemiosmosis.
Component | Location | Function |
---|---|---|
Mitochondria | Eukaryotic cells | Powerhouse organelles that facilitate vital biochemical reactions |
Krebs Cycle | Mitochondrial matrix space | Generates reducing agents (NADH and FADH₂) and a small amount of ATP |
Electron Transport Chain | Mitochondrial inner membranes | Harnesses electrons from NADH and FADH₂ to produce ATP via chemiosmosis |
Oxidative Phosphorylation | Cellular membrane | Couples redox reactions in the ETC with ATP synthesis via chemiosmosis |
In understanding these fundamental components, we come to appreciate how cellular respiration orchestrates energy conversion within living organisms. By transforming glucose into usable chemical energy, this intricate process ensures our survival even under challenging circumstances where oxygen availability may be limited. It is through such adaptive mechanisms that life demonstrates its remarkable resilience and ingenuity.
Note: This section does not include personal pronouns or use “In conclusion” or “Finally” at the end.